Racing electrons under control
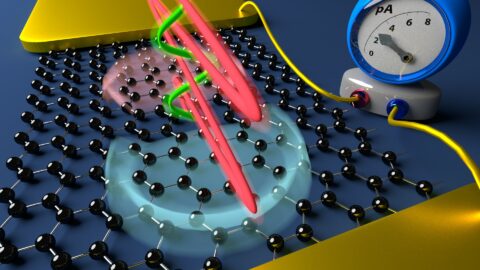
Physicists at FAU precisely control electrons on a time scale of less than one femtosecond
In future, electronics will be controlled via light waves instead of voltage signals: This is the goal of physicists worldwide. The advantage: Electromagnetic waves of light oscillate at the petahertz frequency. This means that future computers could be a million times faster than the current generation. FAU scientists have now come one step closer to this goal: They have succeeded in precisely controlling electrons in graphene with ultra-short laser pulses.
A current control in electronics that is millions of times faster than today: Many dream of it. After all, current control is one of the most important components; it is responsible for data and signal transmission. Controlling electron currents with light waves instead of voltage signals could make this dream come true. But so far it has proved difficult to control electron streams in metals. Because metals reflect light rays, the electrons inside cannot be influenced by the light wave.
FAU physicists therefore resort to graphene: a semi-metal that consists of only one layer of carbon and is therefore so thin that enough light is incident to set electrons in motion. In an earlier study, the physicists from the Chair of Laser Physics had already succeeded in using a very short laser pulse to generate an electrical signal on a time scale of only one femtosecond. This corresponds to the millionth part of a billionth of a second. Under these extreme time scales, electrons reveal their quantum nature: they behave like a wave. Driven by the light field, i.e. the laser pulse, the electron wave glides through the material.
Under control
In the current study, the researchers have gone one step further. They directed a second laser pulse at this light-driven wave. This second pulse allows the electron wave to slide through the material in two dimensions. The second laser pulse can be used to deflect, accelerate or even change the direction of the electron wave. Depending on the exact time of the second pulse, its strength and its direction, information can be transferred to this wave. You can even go one step further: “Imagine the electron wave as a water wave. Water waves can split at an obstacle and interfere when they converge again at the end of the obstacle. Depending on how the two partial waves are related to each other, they can amplify or cancel each other out. With the second laser pulse, we can specifically modify the individual partial waves and thus control their interference,” explains Christian Heide from the Chair of Laser Physics. “In general, it is very difficult to control quantum phenomena such as the wave properties of electrons. This is because it is very difficult to maintain such an electron wave in a material because it scatters with other electrons and loses its wave property. Typically, experiments are carried out at extremely low temperatures. We can now carry out these experiments at room temperature as well, since we can control the electrons via laser pulses so quickly that there is no time at all for scattering processes with other electrons. This will allow us to investigate many new physical processes that were previously inaccessible to us.”
The scientists have thus come a big step closer to electronics controlled by light waves. Over the next few years, they will investigate whether the electrons can also be controlled in other two-dimensional materials. Heide: “Perhaps we can also use materials research to change the properties of the materials so that it will soon be possible to build small light-controlled transistors”.
The scientists have published their results in the journal Physical Review Letters.
Further information:
Prof. Dr. Peter Hommelhoff
Phone: +49-913-/85-27090
peter.hommelhoff@fau.de
Christian Heide
christian.heide@fau.de